Welcome to Design Tips! It is well known that high speed signal traces should not cross a split in the nearest ground-reference plane. However, due to PCB cost/space constraints, we are often forced to route such traces adjacent to power layers, where various power islands exist. If a high speed trace travels from the region of one power island to another, it effectively crosses a split in the nearest reference plane. In this Design Tip, Professor Jun Fan with the Missouri University of Science and
|
Technology discusses how to estimate the impact of this crossing on crosstalk between two traces.
Please send me your most useful design tip for consideration in this section. Ideas should not be limited by anything other than your imagination! Please send these submissions to bruce.arch@ieee.org. I’ll look forward to receiving many “Design Tips!” Please also let me know if you have any comments or suggestions for this section, or comments on the Design Tips articles. |
Crosstalk Estimation for Stripline
Traces Crossing a Split
By Jun Fan, Missouri University of Science and Technology, jfan@mst.edu
In practical printed circuit board (PCB) designs, it is common to split power/ground planes into islands, area fills, or plane portions. Consequently, it may be inevitable to route signal traces crossing a split due to ever-increasing circuit density in design. As a well-known EMC rule of thumb, these traces could potentially cause signal integrity and EMI consequences, and are usually not recommended in practice. One exception is probably the stripline case where only one of the two reference planes is gapped. The hypothesis is that the good reference plane will provide a well-controlled return path, and the discontinuity in the other reference plane has a negligible effect. Studies have demonstrated that these stripline traces do achieve good signal transmission. However, additional crosstalk could be introduced due to the split-crossing. Further, the split could be excited, resulting in potential EMI issues. In this article, we will focus our discussion on crosstalk.
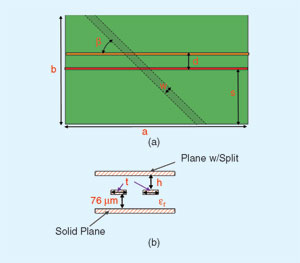 |
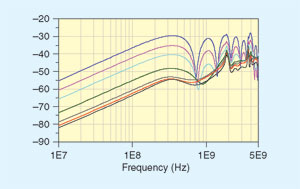 |
Fig. 1. Geometry under study. (a) Top view (b) Cross
section view. |
Fig. 2. |S31| as a function of trace-to-trace separation. |
Let’s look at an example test structure shown in Fig. 1. In this 3-layer structure, two stripline traces are placed in the middle layer. The top layer is partitioned into two parts by a split, while the bottom layer is a solid ground plane. The crosstalk between these two traces is investigated in both the frequency and the time domains.
It is found that the crosstalk between the two stripline traces is a function of the distance between them [1], which agrees with our intuition. For example, Fig. 2 shows the |S31| results in the frequency domain, which represent the near-end crosstalk (NEXT). We can clearly observe the trend in the figure where d is the trace-to-trace separation (in this example, the other geometrical parameters are a = 100 mm, b = 80 mm, s = 40 mm, t = 1 mm,
β = 90°, w = 135 μm, h = 370 μm, and
εr = 4.35). Furthermore, it is noticeable
that the curves can be divided into two categories and they have different shapes. This can be explained as two coupling mechanisms, direct trace-to-trace coupling and split-related coupling, existing in this kind of geometries.
Let’s then decompose the two crosstalk components. The direct trace-to-trace crosstalk can be well estimated using the multi-conductor transmission-line theories assuming both the reference planes are solid. The split-related crosstalk is obtained by subtracting the direct trace-to-trace coupling from the overall crosstalk. The two resulting components are shown in Fig. 3. It can be clearly seen that, when d is smaller than 0.7 mm, the overall S31 magnitude is dominated by the direct trace-to-trace coupling and it increases drastically when d decreases. When d is greater than 0.7 mm, the split-related coupling becomes the dominant noise-coupling mechanism. In these cases, the S31 magnitude does not change with d as significantly as in the cases when the trace-to-trace coupling dominates. The resonances shown in Fig. 3(a) are related to the trace length, and the resonances shown in Fig. 3(c) are related to the split length.
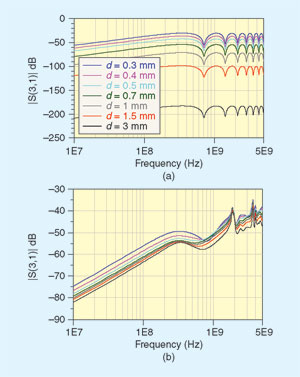 |
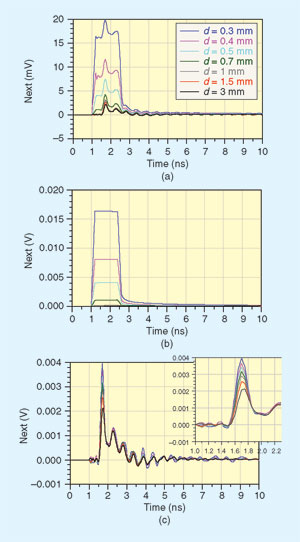 |
Fig. 3. Two crosstalk mechanisms. (a) |S31| due to direct
trace-to-trace coupling (b) |S31| due to split-related coupling. |
Fig. 4. NEXT as a function of trace-to-trace separation.
(a) Overall NEXT (b) NEXT due to direct trace-to-trace coupling (c) NEXT due to split-related coupling. |
The same decomposition procedure can be applied to the NEXT waveforms in the time domain as well. The corresponding results are shown in Fig. 4, where the rise time of the aggressor voltage is 200 ps and both traces are matched at the ends. It can be seen that the direct trace-to-trace coupling decreases quickly as d increases. The split-related NEXT waveform is a single sharp pulse with multiple oscillations. The oscillations are caused by the split-associated resonances. As d increases, the split-related crosstalk decreases too, but not as significantly as the direct trace-to-trace coupling.
By investigating the two crosstalk mechanisms, we can conclude that split-crossing does contribute additional crosstalk among the stripline traces even though one of their reference planes is ideal. Our study shows that we can draw similar conclusions for the FEXT and the NEXT, although only the NEXT results are discussed here for brevity. The split-related crosstalk is relatively weak, but it is not a strong function of the trace-to-trace separation. When two traces are closely located, the crosstalk between them is dominated by the direct trace-to-trace coupling. Otherwise, it is dominated by the split-related coupling. The dimensions of the split contribute to the resonances in the frequency domain and oscillations in the time domain, which could become a trouble maker for sensitive signals when the aggressor signal has large spectral components at these resonant frequencies.
Using this effective decomposition technique, we can further study the split-related crosstalk as a function of various geometrical dimensions. In addition to the trace-to-trace separation, the trace-to-split-plane distance and the rise/fall time of the aggressor signal are found to be the other two dominant factors. When the traces move closer to the split plane (h decreases), both the NEXT and FEXT magnitudes increase. This makes sense since, as h decreases, more return current flows in the split plane, resulting in a higher level of split-related crosstalk. The split-related crosstalk also increases with the decrease of the rise/fall time of the aggressor signal. And this increase is more significant when the rise/fall time is smaller. The other geometrical parameters, such as the split length b, the aggressor-to-board-edge distance s, the split width w, the split-trace angle β, the trace width t, and the dielectric constant εr, do not play significant roles in the split-related crosstalk.
STAY TUNED!
“By investigating the two crosstalk mechanisms, we can conclude that split-crossing does contribute additional crosstalk among the stripline traces even though one of their reference planes is ideal. Our study shows that we can draw similar conclusions for the FEXT and the NEXT, although only the NEXT results are discussed here for brevity.” The FEXT results will be discussed in the next, Summer 2011, issue of the EMC Newsletter. Stay tuned! |
Lastly, but certainly not the least, what happens when there are multiple aggressors? Fortunately, our study shows that superposition holds. In other words, if multiple traces cross a split, the overall crosstalk voltage on the victim line can be estimated simply by adding up the crosstalk from each individual aggressor. This implies that using differential signaling can effectively reduce the split-related crosstalk, although the cancellation will not be complete due to the asymmetry of the geometry.
Reference
[1] S. Wu, M. Herndon, H. Shi, B. Cornelius, and J. Fan, “Crosstalk among multiple stripline traces crossing a split,” DesignCon 2011, Santa Clara, CA, January 31–February 3, 2011. EMC
|