
April 6, 2010
Ray, Beam and Hybrid
Techniques for
Analysis of Electrically Large Antenna Configurations
Professor Prabhakar H. Pathak
At sufficiently high
frequencies,
for which radiating objects become quite large (or even moderately
large) in
terms of the wavelength, conventional numerical methods employed for
the
solution of practical antenna radiation problems become strained or
even
intractable. It then becomes natural and far more efficient to employ
asymptotic high frequency ray and beam methods, and also hybrid methods
which
combine high frequency and numerical techniques, respectively, to
analyze
electrically large antenna problems. A uniform version of the
geometrical
theory of diffraction (GTD) [ 1 ], which is referred to as the UTD [
2,3 ], is
one such ray method which can be used effectively. A significant
advantage of
the UTD is that it offers a physical insight into the antenna radiation
mechanisms involved in terms of diffracted rays together with
conventional
geometrical optics (GO) rays. Such a direct and vivid picture based on
a ray
description of the radiation of electromagnetic (EM) waves is typically
not
shared by any other methods of solution. The power of UTD will be
illustrated
through applications to antennas in complex environments. In some
special
situations involving a confluence of ray caustics (or foci) with ray
shadow
boundaries the UTD alone may become inapplicable. In the latter
instance, the
high frequency physical optics (PO) method is often employed; however,
PO
generally needs an integration of the assumed currents on the large
radiating
object thus making it rapidly inefficient with increase in the
operating
frequency. A useful and more accurate alternative is the use of beam
methods,
e.g., the one based on the complex source beam (CSB) approach [4,5,6].
An
extension of the UTD for CSB illumination is developed and will be
described
for specific applications. In many practical applications, a radiating
object
can contain both electrically large and small parts; in such cases it
becomes
necessary to hybridize high frequency and numerical methods in order to
combine
the best features of both methods and hence also to overcome the
limitations of
both. In particular, examples involving the application of UTD, CSB,
and hybrid
methods, respectively, will be presented highlighting the power of
each.
Examples will include the treatment of large satellite antenna
reflector
systems, and other antennas including large complex and conformal
phased arrays
on complex airborne, space borne or ship platforms, and of antennas in
urban/rural environments. In addition, an analysis of near field
antenna
measurements for far zone pattern predictions will also be considered.
June
20, 2010
ULTRA-WIDEBAND
AND INTERLEAVED
POLYFRACTAL ANTENNA ARRAYS
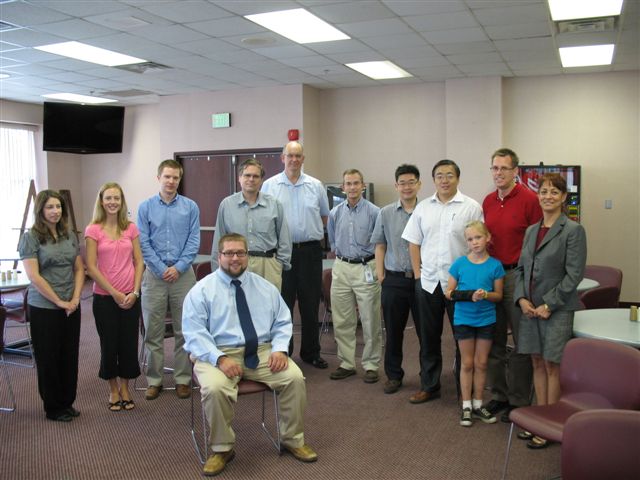
November 18, 2010
Time Domain Finite-Element
Finite-Difference
Hybrid Method and Its Application to Electromagnetic Scattering and
Antenna
Design
Shumin
Wang .